User:Nitech2008/Oscillatory baffled reactor

![]() | The copyright holder of this file has irrevocably released all rights to it, allowing it to be freely reproduced, distributed, transmitted, used, modified, built upon, or otherwise exploited in any way by anyone for any purpose, commercial or non-commercial, with or without attribution of the author, as if in the public domain. However, as a courtesy, a link back to [http://www.nitechsolutions.co.uk would be appreciated. |

![]() | The copyright holder of this file has irrevocably released all rights to it, allowing it to be freely reproduced, distributed, transmitted, used, modified, built upon, or otherwise exploited in any way by anyone for any purpose, commercial or non-commercial, with or without attribution of the author, as if in the public domain. However, as a courtesy, a link back to [http://www.nitechsolutions.co.uk would be appreciated. |
There has been a significant demand in the chemical and pharmaceutical industries for the more efficient use of reagents, solvents and energy while minimising the production of out-specs and waste materials. These needs have led to a number of continuous reactors being developed in recent years, and oscillatory baffled reactor (OBR) is one such a reactor. The OBR technology generally consists of a cylindrical column or tube containing equally spaced orifice baffles and superimposing with fluid oscillation. Eddies are generated when fluid flow passes through the baffles as shown in Figure 1, enabling significant radial motions where events at the wall are of the same magnitude as these at the centre.[1] The generation and cessation of eddies creates uniform mixing in each baffled cell, collectively along the column or tube.
The OBR is related to batch and fed-batch operations, and generally operated vertically. In OBR, the oscillation can be achieved by either using a piston or bellows arrangement at the base of the column or moving a set of baffles up and down the column at the top. When there is no reaction involved in an OBR, the term of oscillatory baffled column (OBC) is often used.
By connecting baffled cells in series, a continuous oscillatory baffled reactor (COBR) is created, as shown in Figure 3. As each baffled cell acts as a continuous stirred tank reactor (CSTR), with a large number of baffled cells in a COBR plug flow condition is achieved under laminar flow (low flow rates). The plug flow characteristics of residence time distribution (RTD) in a COBR is illustrated in Figure 4.

![]() | The copyright holder of this file has irrevocably released all rights to it, allowing it to be freely reproduced, distributed, transmitted, used, modified, built upon, or otherwise exploited in any way by anyone for any purpose, commercial or non-commercial, with or without attribution of the author, as if in the public domain. However, as a courtesy, a link back to [http://www.nitechsolutions.co.uk would be appreciated. |

![]() | The copyright holder of this file has irrevocably released all rights to it, allowing it to be freely reproduced, distributed, transmitted, used, modified, built upon, or otherwise exploited in any way by anyone for any purpose, commercial or non-commercial, with or without attribution of the author, as if in the public domain. However, as a courtesy, a link back to [http://www.nitechsolutions.co.uk would be appreciated. |
The COBR is for the continuous processing and operations, and can be operated horizontally, vertically or at any angle. The key differences between the COBR and any other tubular devices on the market are:
a) Netflow (rate) is the sole driving source for achieving mixing in all other tubular devices on the market, accommodating very short reaction times, e.g. 15 mins max. The mixing in COBR however is governed by the combination of fluid oscillation and the presence of baffles, not the net flow, allowing significantly longer residence time (in terms of hours);
b) As mixing is controlled by the combination of baffles and oscillation, plug flow conditions are achieved under laminar flow (low flow rates) in COBR. This is not possible for any other tubular devices on the market;
c) Due to the attainment of plug flow under laminar flow conditions in COBR, much more compact reactor design and configurations are resulted with significant reduction (>90%) of reactor volume/space. The COBR is portable and a useful reactor for lean manufacturing;
d) Much reduced pressure drop is realised in COBR due to the low flow rates;
e) COBR is very good in handling solids, which is an unique property of COBR and differentiates from arrays of micro/meso flow devices;
f) Significantly enhanced mass and heat transfers are obtained in COBR> under plug flow.
When solid particles are present in a liquid phase, uniform and efficient suspension and transportation of solids is obtained along the length of COBR.[2] When a gas phase is involved, very good mixing and dispersion of the gas into the liquid occurs to give an exceptionally high surface contact area and a substantial increase in gas hold up and gas residence time, enabling enhanced mass transport characteristics.[3]. Due to plug flow conditions achievable in COBR, it also offers superior heat transfer rate.[4] Note that the connections between the straight baffled tubes and bends as shown in Figure 3 can be used for inputting, sampling, monitoring and outputting. The jackets along the COBR provide heating/cooling in either an individual or integrated manner so that different or same thermal profiles can be established along the length of the COBR.
Description
[edit]The oscillatory baffled reactor is generally understood to be a cylindrical tube or column containing periodically spaced orifice baffles in which a liquid or multiphase fluid is oscillated axially by means of diaphragm, bellows or piston at one or both ends of the tube. For batch operation, such a column is run vertically and fluid oscillation can be achieved either at the base by means of bellows or at the top by moving a set of orifice baffles up and down the column. The mechanism of mixing in such an OBR can be understood with the help of Figure 5.

![]() | The copyright holder of this file has irrevocably released all rights to it, allowing it to be freely reproduced, distributed, transmitted, used, modified, built upon, or otherwise exploited in any way by anyone for any purpose, commercial or non-commercial, with or without attribution of the author, as if in the public domain. However, as a courtesy, a link back to [http://www.nitechsolutions.co.uk would be appreciated. |
The essential feature is that sharp edges (provided by the baffles) are presented transverse to an oscillating and fully reversing flow. The periodic motion of the flow accelerates and decelerates according to a sinusoidal velocity-time function. On each flow acceleration, vortex rings form downstream of the baffles. When the flow decelerates, those vortices are swept into the bulk, and subsequently unravelled with the bulk flow acceleration in the opposite (axial) direction.
With the repeating cycles of vortex formation, the strong radial velocities give uniform mixing in each inter-baffle zone and cumulatively along the length of the column.[5][1] The key feature of OBR is that mixing can be controlled to a very high degree of precision, giving a wide range of mixing conditions, from “soft” mixing, exhibiting plug flow characteristics, to the most intense, approaching mixed flow conditions.
The fluid mechanical conditions in an OBR are generally governed by two dimensionless numbers: the oscillatory Reynolds number (Reo = 2πfDxo/μ)
and the Strouhal number (St = D/(4πxo)),
where D is the column diameter (m), ρ the fluid density (kg/m3), μ the fluid viscosity (kg/ms), xo the oscillation amplitude (m) and f the oscillation frequency (Hz). The oscillatory Reynolds number describes the intensity of mixing applied to the column, while the Strouhal number is the ratio of column diameter to stroke length, measuring the effective eddy propagation.[6]
Plug Flow
[edit]The term of plug flow is such that the radial mixing is uniform, and the axial dispersion is of a minimum.[7] Plug flow ensures consistent fluid mechanical conditions with no gradient in mixing and heating/cooling, leading to consistent product qualities. It is worth mentioning that plug flow can ONLY be achieved in continuous operation. There are two textbook methods of achieving plug flow: a) to use a series of continuous stirred tank reactors (CSTR), and b) to employ a tubular reactor operating at turbulent flow. The first method is literately to connect a number of well-stirred tanks of equal volume in series. In theory, when the number of CSTR goes to infinite, plug flow is achieved. In practice, 5~10 CSTRs have been the norm of most industries today! This arrangement significantly increases inventory in reactors with multiples of controllers, pressure sensors, probes, pumps, flow meters, etc, leading to significantly high capital and running costs; not to mention that the fluid mechanical conditions are still far from plug flow.
The second method is to employ a smooth tubular reactor with or without inserts, which is operated at turbulent flow, i.e. very high flow rates at a given tube diameter. When the turbulent flow is reached, near to plug flow conditions can be obtained. However, as the mixing in such tubular reactors is driven by the net flow, this means that a very long tube (in terms of miles) is required even to accommodate a short reaction! Hence, such an operation is practically impossible. As a consequence of this, to date batch STR have been the workhorse of chemical and pharmaceutical industries.
The most significant advantages for COBR are its ability of achieving plug flow under laminar flow conditions and its residence time distribution (RTD) performance can be improved independently of net flow conditions; as well as its capability of handling multiphase processes.[8][1][9][10] This makes COBR ideal for use as plug flow reactors in chemical, pharmaceutical, food and drinks or biochemical industries, while maintaining long residence times where other tubular devices fail to achieve.
Heat Transfer Enhancement
[edit]Superimposing an oscillatory flow onto a steady flow through a baffled tube can significantly enhance heat transfer in batch and continuous operation. In particular, the tube-side heat transfer coefficient (Nusselt number), where the mean flow is in the laminar flow regime, is typically enhanced by a factor of 10-30.[11] This makes it possible to achieve heat transfer coefficient of the same order as in smooth tubes where significantly high steady flow Reynolds numbers are used to obtain turbulence. In practice, this means that high heat transfer coefficient can be achieved in continuous reactors using oscillatory flows, at considerably lower Reynolds numbers than in conventional continuous tubular reactors.
The following provides an indication of the range of heat transfer coefficient that can be obtained using the COBR.
Figures are based on:
Cp = 2000 kJ/kgK and k = 0.182 W/mK
Tube side heat transfer coefficient, ht (W/m2K)
1 cP | 10 cP | 100 cP | |
---|---|---|---|
600 kg/m3 | 61,654 - 282,717 | 817 - 3,694 | 7 - 28 |
1000 kg/m3 | 133,315 - 611,447 | 2,327 - 10,575 | 21 - 82 |
1500 kg/m3 | 232,744 - 1,067,534 | 5,209 - 23,730 | 47 - 196 |
Within the range of figures indicated, mixing intensity (amplitude and frequency) is the key factor that affects the actual heat transfer rate.
Crystallisation
[edit]Crystallisation is one of the oldest unit operations and is widely used in fine & speciality chemicals and pharmaceutical industries. Almost 8 out of 10 pharmaceutical products and 6 out of 10 fine and speciality chemicals are produced via a crystallisation methodology.[12] Examples of products include antibiotics, cancer drugs, healthy food ingredients, food additives, beauty products, and personal products, to name but a few. These products can be highly complex and in large manufacturing plants are often difficult to process to the standards demanded by consumers and regulators.
Challenges in industrial batch crystallisation
[edit]A number of process variables affect crystallisation process: supersaturation, solute concentration, temperature, mixing, cooling profile, solvent/additives and seeding. Since no complete theory is available to model nucleation/crystallisation, their behaviors can only be anticipated by experimentation; process measurements are essential in understanding crystallisation processes. A number of techniques have been implemented to monitor crystallisation/process variables at lab scale, for example, employing optical turbidometric UVvis probe for the measurement of metastable zone width;[13] X-ray diffraction (XRD) for polymorph;[14] Fourier transform infrared spectroscopy (FTIR) for supersaturation;[15] ultra sound spectroscopy (USS) for crystal size;[16] focused beam reflectance measurement (FBRM) for on-line chord length of crystals and crystal size distribution;[17] particle vision measurement (PVM) for on-line crystal shape and particle image velocimetry (PIV) for local velocity, etc. These techniques/measurements have promoted significant advances in understanding lab scale batch crystallisation and have assisted in designing better crystallisation processes.
However, these advances have not been matched by an equivalent increase in our ability and understanding in scaling up stirred tank reactors (STR), the workhorse of industrial crystallisation. The scale-up of crystallisation processes involves a net result of several independent, but interrelated, steps. A crystallisation vessel is three-dimensional, so as the linear dimensions increase, the capacity of the system increases as the cube of the linear dimension. With this increase in scale, other variables rise on the linear scale with different exponents, which may vary from negative to zero to three and higher. As a result, the scale-up of STRs has always been very troublesome. In addition, there is no agreement on the set of parameters to be kept constant in scale-up. This has led to some of the problems that have existed in industrial crystallisation processes today: very long heating and cooling cycles, due to the huge batch mass/volumes involved; large concentration and temperature gradients in plant scale crystallisers due to the non-uniform mixing and large velocity gradients, making the required “concentration-temperature path” uncontrollable.[18] Inconsistent crystal morphology, long filtration time and inconsistent product quality are some of the direct outcomes of these problems.
In addition, the techniques aforementioned provide local measurements, i.e. events at either a point or a very small area within a given crystallisation vessel. The principle of such measurements is based on the assumption that the vessel is well mixed, so that local events can be used to represent the overall measurement of the whole system. At lab and small scales, such an assumption may well be valid; however, both the confidence and capability of measuring techniques decrease substantially with the increase in scale. As a result, instrumentation that has widely been used in lab and small scale crystallisers is seldom found in batch industrial crystallisation. Even when some measuring probes are employed in large scales, the location of these probes has to carefully be selected, otherwise, the measurements might not be wholly representative or, the local fluid mechanical conditions are so poor that the faces of probes are fully covered or in some cases stuck by crystals, making measurements impossible. Furthermore, a simple task, such as, implementing some of the aforementioned measurement equipment in industrial scale crystallisation is still far from practical.
From the operational viewpoint, the linear cooling profile, i.e. a linear relationship between cooling temperature and time, has been identified as one of the key parameters to achieve the “concentration-temperature path” in solution crystallisation. Whilst linear cooling profile is routinely used in laboratories, it is still impossible to be implemented in any industrial scale batch STR due to its huge volume, inherited non-uniform mixing and significant concentration/temperature gradients. Clearly, there is still a gulf between the laboratory understanding and industrial-scale crystallisation; the benefits being realised in laboratory STR cannot be translated into the large industrial operations.
Continuous oscillatory baffled crystalliser (COBC)
[edit]
![]() | The copyright holder of this file has irrevocably released all rights to it, allowing it to be freely reproduced, distributed, transmitted, used, modified, built upon, or otherwise exploited in any way by anyone for any purpose, commercial or non-commercial, with or without attribution of the author, as if in the public domain. However, as a courtesy, a link back to [http://www.nitechsolutions.co.uk would be appreciated. |
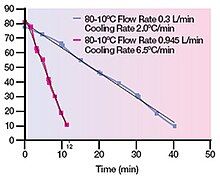
![]() | The copyright holder of this file has irrevocably released all rights to it, allowing it to be freely reproduced, distributed, transmitted, used, modified, built upon, or otherwise exploited in any way by anyone for any purpose, commercial or non-commercial, with or without attribution of the author, as if in the public domain. However, as a courtesy, a link back to [http://www.nitechsolutions.co.uk would be appreciated. |
Combining the COBR technology with synchronisation of both global and local temperature controls, a continuous oscillatory baffled crystalliser (COBC) is established. Figure 6 is a snapshot of the set up of a COBC. The COBC allows both plug flow and precise linear cooling profiles, in terms of either oC/min or oC/m, along the length of the COBC. Figure 7 is one of the typical examples of linear cooling profiles achievable in COBC, e.g. cooling linearly from 80 to 10 oC over 40 minutes at a cooling rate of 0.3 oC/min and from 80 to 10 oC about 10 minutes at a cooling rate of 6.5 oC/min. In fact, with the set up of COBC, any form/function of cooling profiles can easily be implemented, and profiles can be linear, non-linear, continuous, stepwise or discontinuous. In addition, any of the aforementioned measuring instruments can easily be implemented along the COBC without any modification, facilitating smooth knowledge transfer from lab to full scales. As the mixing in each baffled cell along the COBC is uniform, even at industrial scales where the diameter of COBC is seldom greater than 150 mm, it provides a highly reliable environment for process monitoring and measuring; the local measurements can be used to represent the whole system with significantly increased confidence. An example of continuous crystallisation of active pharmaceutical ingredient (API) is reported where the crystallisation time was reduced from 9 hours and 40 minutes in batch STR operation to 12 minutes in continuous operation [19].
The UK Engineering and Physical Sciences Research Council (EPSRC)Centre for Innovative Manufacturing provides a national focus for Continuous Manufacturing And Crystallisation CMAC[20] with GSK, AstraZeneca and Novartis as the funding members plus over 100 PhD students in seven UK universities working on a large variety of subjects from continuous work up to crystallisation, from continuous drying to tabletting and from strategies to logistics.
Mass Transfer Enhancement
[edit]
![]() | The copyright holder of this file has irrevocably released all rights to it, allowing it to be freely reproduced, distributed, transmitted, used, modified, built upon, or otherwise exploited in any way by anyone for any purpose, commercial or non-commercial, with or without attribution of the author, as if in the public domain. However, as a courtesy, a link back to [http://www.nitechsolutions.co.uk would be appreciated. |

![]() | The copyright holder of this file has irrevocably released all rights to it, allowing it to be freely reproduced, distributed, transmitted, used, modified, built upon, or otherwise exploited in any way by anyone for any purpose, commercial or non-commercial, with or without attribution of the author, as if in the public domain. However, as a courtesy, a link back to [http://www.nitechsolutions.co.uk would be appreciated. |
When a gas phase is involved in an OBR, very good mixing and dispersion of the gas into the liquid occurs to give an exceptionally high surface area due to uniform and even gas bubble size distribution and a substantial increase in gas hold up and gas residence time, leading to significantly enhanced mass transfer coefficient.[3] Figure 8 compares the concentrations of a biopolymer fermented in a batch OBR and STR. It is clear that flow oscillations increase mass transfer rate.
The applications of OBR in liquid-gas processes include: hydrogenation[21], oxidation, hydroformlytion. Figure 9 is the 100 bar OBR hydrogenator.
Reduction of Emission
[edit]
![]() | The copyright holder of this file has irrevocably released all rights to it, allowing it to be freely reproduced, distributed, transmitted, used, modified, built upon, or otherwise exploited in any way by anyone for any purpose, commercial or non-commercial, with or without attribution of the author, as if in the public domain. However, as a courtesy, a link back to [http://www.nitechsolutions.co.uk would be appreciated. |
Most organic syntheses involve multistage reactions. As each stage can take a number of hours, the overall reaction time can be very long in terms of days or weeks. In industrial operations, this overall reaction time generally consists of the intrinsic and the external reaction times. The latter largely results from mass, heat gradients built up in a given batch reactor due to non-uniform mixing, and is often significantly longer than the intrinsic reaction time that is referred in reaction engineering textbooks. The mass/heat gradients have two effects: they give rise to a strong mass transfer-controlled reaction process; and they slow down the main reaction, which in turn encourages side reactions. The removal of the mass/heat gradients in a COBR ensures optimal reaction conditions for reactants to react at the correct concentration and temperature, hence reduces unwanted side reactions and emissions. Figure 10 shows NOx emissions in the production of a speciality chemical in both a batch STR and a COBR. The plug flow COBR completely eliminated the emission of NOx.
Control of Shear Rate
[edit]It has been shown that the volume averaged shear rate in OBR is generally of order 10~20 /s, which is significantly lower than that in stirred tank reactors (STR), which are typically at least 100 /s or greater, while the mixing in OBR is more uniform and efficient than that in STR. The production of a cellular biopolymer, pullulan, in OBR shows that it took about half the time (36 hrs) in the OBR to achieve the same level of growth as compared to that in a STR for the same operational conditions.[22] This feature of good global mixing coupled with low and uniform shear rate is essential to shear-sensitive applications in biochemical, biomedical and biopharmaceutical processes.
Commercialisation
[edit]NiTech® Solutions Ltd is a commerical company who applies OBR, COBR, COBC and TBR mixing technologies to various chemical and pharmaceutical processes with continuous crystallisation being one of its core businesses. Table below lists the range of proven multiphase processes carried out by NiTech®.
PHASES | REACTIONS | FEASIBILITY COMPLETED | CONTINUOUS PILOT PLANT COMPLETED | |
---|---|---|---|---|
TBRTM |
Liquid-Solid-Gas Liquid-Liquid-Solid Liquid-Solid Liquid-Liquid |
Carbonation Hyaluronic acid Blending Formulation Acetylation Basification |
Yes for all |
Yes for all |
COBRTM |
Liquid-Solid-Gas Liquid-Solid
Liquid-Liquid
Liquid-Gas
Liquid-Liquid separation |
Hydrogenation Wetting Diazotisation Cyclisation Drug encapsulation Acetylation Bromination Enzymatic reactions Polymer dissolution Polymerisation Transesterification Hydration of nitrile amide Boranation Nitration Coagulation Ene reaction Oxidation Hydroformylation In-line phase separation |
Yes for all
|
Yes Yes Yes Yes Yes
Yes Yes Yes
Yes Yes Yes |
COBCTM |
Cooling crystallisation Anti-solvent crystallisation Oil fractionation |
Pharma APIs Fine chemicals Speciality chemicals Bulk chemicals Edible oils |
Yes for all
|
Yes Yes Yes Yes |
Some of NiTech®'s industrial applications are also summarised in the following photos, and you can find out more details from http://nitechsolutions.co.uk.

![]() | The copyright holder of this file has irrevocably released all rights to it, allowing it to be freely reproduced, distributed, transmitted, used, modified, built upon, or otherwise exploited in any way by anyone for any purpose, commercial or non-commercial, with or without attribution of the author, as if in the public domain. However, as a courtesy, a link back to [http://www.nitechsolutions.co.uk would be appreciated. |

![]() | The copyright holder of this file has irrevocably released all rights to it, allowing it to be freely reproduced, distributed, transmitted, used, modified, built upon, or otherwise exploited in any way by anyone for any purpose, commercial or non-commercial, with or without attribution of the author, as if in the public domain. However, as a courtesy, a link back to [http://www.nitechsolutions.co.uk would be appreciated. |

![]() | The copyright holder of this file has irrevocably released all rights to it, allowing it to be freely reproduced, distributed, transmitted, used, modified, built upon, or otherwise exploited in any way by anyone for any purpose, commercial or non-commercial, with or without attribution of the author, as if in the public domain. However, as a courtesy, a link back to [http://www.nitechsolutions.co.uk would be appreciated. |

![]() | The copyright holder of this file has irrevocably released all rights to it, allowing it to be freely reproduced, distributed, transmitted, used, modified, built upon, or otherwise exploited in any way by anyone for any purpose, commercial or non-commercial, with or without attribution of the author, as if in the public domain. However, as a courtesy, a link back to [http://www.nitechsolutions.co.uk would be appreciated. |

![]() | The copyright holder of this file has irrevocably released all rights to it, allowing it to be freely reproduced, distributed, transmitted, used, modified, built upon, or otherwise exploited in any way by anyone for any purpose, commercial or non-commercial, with or without attribution of the author, as if in the public domain. However, as a courtesy, a link back to [http://www.nitechsolutions.co.uk would be appreciated. |
Limitations
[edit]Every new technology on the market has its limitations, so have the OBR, COBR and COBC. Based on the experiences and real industrial projects carried out using the above reactor/crystalliser technologies, the following is the summary of these imperfections:
a) As gas dampens the propagation of fluid oscillation, hence the degree of mixing, along the above reactors, the amount of gas/gases in liquid/liquids should be determined. In theory, about 15% (v/v) would be the maximum allowance for these reactors. As a result, the COBR reactors would not be suited for reactions with their final products as gas, such as ethylene, propene and methylene or with one of the main reactants being a gas phase, e.g. production of ethylene glycol using ethylene oxide.
For majority of the liquid based organic syntheses and reactions, the maximum allowance could be exceeded by recycling the product liquid stream.
b) Too many solids would affect the propagation of oscillation and mixing in these reactors. In theory, about 25% (w/w) would be the upper ceiling depending on particle size distribution, density and etc.
c) Viscosity of liquid or a mixture of liquids is the 3rd factor for consideration, as fluid oscillation cannot effectively spread through viscous liquid/liquids. In theory, main fluid with viscosity exceeding 500 cP would not be suitable for these above reactors. However, as most liquids used in chemical and pharmaceutical industries are Newtonian liquids with their viscosities decreasing with the increase of temperature, the limit would go up when operating at elevated temperature.
See also
[edit]- Crystallization
- Plug flow reactor
- Pharmaceutical industry
- Chemical industry
- Lean manufacturing
- Chemical reactor
- Organic synthesis
- Bioprocessing
References
[edit]- ^ a b c M.R. Mackley and X. Ni, “Mixing and dispersion in a baffled tube for steady laminar and pulsatile flow”. Chemical Engineering Science, Vol. 46, No. 12, 1991, 3139-3151.
- ^ [1]
- ^ a b M.S.N. Oliveira and X. Ni, “Effect of hydrodynamics on mass transfer in a gas-liquid oscillatory baffled column”, the Chemical Engineering Journal, Vol. 99, 2004, 59-68.
- ^ [2]
- ^ Brunold, C.R., Hunns, J.C.B., Mackley, M.R and Thompson, J.W. (1989). Experimental observations on flow patterns and energy losses for oscillatory flow in ducts containing sharp edges, Chem. Eng. Science, 44, p1227-1244.
- ^ X. Ni and P. Gough, “On the discussion of the dimensionless groups governing oscillatory flow in a baffled tube”. Chemical Engineering Science, Vol. 52, No. 18, 1997, 3209-3212.
- ^ X. Ni, “Unwrapping the myth about plug flow”, the Chemical Engineer, issue 779, 2006, 26-28.
- ^ Dickens, A.W., Mackley, M.R. and Williams, H.R., 1989, Experimental residence time distribution measurements for unsteady flow in baffled tubes. Chem. Eng. Sci. 44: 1471-1479.
- ^ Stonestreet, P. and van der Veeken, P.M.J., 1999, The effects of oscillatory flow and bulk flow components on the residence time distribution in baffled tube reactors. Trans IChemE, Part A, 77: 671-684.
- ^ Ni, X. and Pereira, N., 2000, Parameters affecting fluid dispersion in a continuous oscillatory baffled tube. AIChE J., 46(No. 1): 37-45.
- ^ Mackley, M.R., Tweedle, G.M. and Wyatt, I.D., 1990, Experimental heat transfer measurements for pulsatile flow in baffled tubes. Chem. Eng. Sci., 45: 1237-1242.
- ^ GSK, Crystallisation in pharmaceutical industry, The British Association for Crystal Growth annual meeting, Leeds University, 2004.
- ^ Hennessy, A., Neville, A. and Roberts, K.J. In-situ SAXS/WAXS and turbidity studies of the structure and composition of multi-homologous n-alkane waxes crystallised in the absence and presence of flow improving additive species, Crystal Growth and Design, 2004, 4, p1069-1078.
- ^ Lai, X., Roberts, K.J., Bedzyk, M.J., Lyman, P.F., Cardoso, L.P. and Sasaki, J-M. Structure of habit modifying trivalent transition metal cations (Mn3+, Cr3+) in nearly perfect single crystals of potassium dihydrogen phosphate (KDP) as examined by X-ray standing waves, X-ray absorption spectroscopy and molecular modelling, Chemistry of Materials, 2005, 17, p4053-4061.
- ^ Borissova, A., Dashova, Z., Lai, X. and Roberts, K.J. Examination of the semi-batch crystallisation of benzophenone from saturated methanol solution via aqueous anti-solvent drowning-out as monitored in-process using ATR FTIR spectroscopy, Crystal Growth and Design, 2004, 4, p1053-1060.
- ^ Mougin, P., Wilkinson, D. and Roberts, K.J. In situ ultrasonic attenuation spectroscopic study of the dynamic evolution of particle size during solution-phase crystallization of urea, Crystal Growth & Design, 2003, 3, p67–72.
- ^ Rousseau, R.W. and Barthe, S. Using FBRM Measurements, Fines Destruction and Varying Cooling Rates to Control Paracetamol CSD in a Batch Cooling Crystallizer, the 2005 Annual Meeting of AIChE, Cincinnati, USA, 2005.
- ^ X. Ni, “Continuous crystallisation using oscillatory baffled crystalliser is the key to bridge the gap between lab and industrial crystallisation”, the Chemical Engineer, issue 800, February 2008, 36-38.
- ^ S. Lawson, G. Steele, P. Shering, I. Laird, L. Zhao and X. Ni, “Continuous crystallisation of pharmaceuticals using a continuous oscillatory baffled crystalliser”, Organic Process Research & Development, Vol. 13, 2009, 1357-1363.
- ^ [3]
- ^ [4]
- ^ H.K. Gaidhani, B. McNeil and X. Ni, “Fermentation of pullulan using an oscillatory baffled bioreactor”, the Chemical Engineering Research and Design, Vol. 83(A6), 2005, 640-645.