User:Awebb6/sandbox/Titantium in zircon geothermometry
Zircon Titanium Geothermometry
[edit]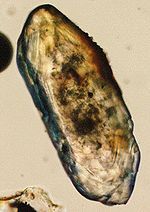
Zircon Titanium Geothermometry, or "Ti-in-zircon Geothermometry" is a process by which the crystallization temperature of a zircon crystal can be determined by the amount of titanium atoms found in the crystal lattice. Zircon is a useful mineral due to its abundance in the Earth's crust, its ability to incorporate various radioactive and nonradioactive isotopes, its durability, and its resistance to isotopic diffusion.[1] In zircon crystals, Titanium is commonly incorporated, replacing similarly charged Zr and Si atoms. This process is relatively unaffected by pressure and highly temperature dependent, with the amount of Ti incorporated rising exponentially with temperature,[1][2] making this an accurate geothermometry method. This method is extremely useful, as it can be combined with radiometric dating techniques that are commonly used with zircon crystals (see Uranium-lead dating), to give quantitative temperature measurements at specific absolute ages through the process known as Geothermochronology.
Zircon
[edit]

Zircon ((Zr1–y, REEy)(SiO4)1–x(OH)4x–y)) is and orthosilicate mineral that commonly found as an accessory mineral throughout Earth's crust.[3]
Zircon is a particularly useful mineral because of its ability to incorporate many trace elements. It is know to exchange Rare Earth Elements (REE) such as Hafnium, Uranium, Thorium, Yttrium, and Lutetium. However, the chemical potential energies of these REE substitutions are not well understood, so they are not suitable for determining crystallization temperatures. Titanium is also incorporated into zircon, and its exchange rates has been studied in detail. Ti4+, a tetravalent ion, can replace Zr4+ or Si4+ in a temperature dependent mechanism. For zircons in the presence of TiO2, i.e. the mineral rutile, this substitution process is common and can be measured.[1]
Thermally, zircon is resistant to temperature changes and extremes. It is stable up to 1690° C at ambient pressure and has a low thermal expansion rate. Zircons crystals are also some of the most incompressible silicate minerals.[3]
Zircons are know to be relatively retentive of their incorporated isotopes and thus very useful for microquantitative studies. Cations such as REE,[4] U, Th, Hf,[5] Pb,[6] and Ti[7] diffuse slowly out of zircons, and their measured quantities in the mineral are diagnostic of the cooling conditions of the crystal.
Methods
[edit]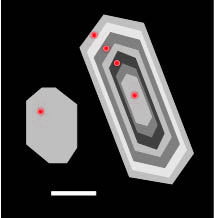
Once a rock has been collected, zircons are extracted using a series of techniques such as using a sieve, heavy liquid, shaking table, and magnetic separation to separate minerals based on differing densities and properties. Zircon crystals are then mounted to an epoxy or metal disc-shaped slide,[9] where they can be shaved to about half thickness to reveal their internal structure. From here, they can be imaged using cathodoluminescence to make any zonations in the mineral visible. If zonation is apparent, multiple measurements of Ti abundance can be taken from the center to the rim to give the temperature evolution of the crystal.
The final step involves measuring the abundance of titanium in a specific location on a zircon crystal with an ion microprobe. For this, the chemical compositions of the zircons is measure using secondary ion mass spectrometry. The sample is bombarded with a beam of primary ions, and the charge and mass of the ejected secondary ions are measured to determine the chemical composition at the point of contact. The quantitative value for titanium content is then compared to a known relationship of titanium incorporation and temperature to determine the crystallization temperature of that zone of the zircon. The titanium-to-temperature relationship was calculated using in situ radiometrically dated zircons with known melt temperatures from the surrounding rock. This Ti-in-zircon measurement can be done several times in zoned zircons, which may record the temperature evolution that resulted from many geologic events.[1][2][10]
Uses
[edit]Geothermometry techniques can provide evidence for changes in temperature in various environments, the thermodynamic evolution of rocks, the gradual change in the geothermal gradient over geologic time, and determine provenance of detrital sediments. Coupled with geochronology techniques using radiometric decay and dating, such as with U/Pb decay, these paleotemperature measurements can be paired with an absolute age in order to determine temperature changes over time.
Ti-in-zircon geothermometry has so far been used to infer cooling temperatures in Hadean age rocks (>4.0 Ga). Low crystallization temperatures from zircons of this age suggests the Hadean Earth contained liquid water, which reduced the cooling temperature of crustal materials. [2] Future studies using Ti-in-zircon thermometry can show the progressive heat loss from a magmatic Hadean Earth, to the onset of plate tectonics as the planet's crust begins to cool and deform.
On a more recent timescale, Ti-in-zircon geothermometry can be used in zircons found in sedimentary rocks to help determine the source of these detrital minerals. However, these crystals may sometimes be contaminated by external titanium seeping into fractures.[1]
Errors and Limitations
[edit]Ti-in-Zircon geothermometry is considered to be a relatively reliable and accurate method of determining crystallization temperatures of zircons, with a error of only 10-16 degrees Celsius. However, the major constraint of this process is that it is only usable in systems that contain rutile (TiO2). In systems that have no or very little titanium, this method is pointless. [2]
Another difficulty with this microanalysis is the contamination of Ti on external surfaces. Recent studies have expressed concern over the gold coating on the surface of the ion microprobe mounts, which contains Ti (~1 ppm), and the Ti-bearing oxide coating on the surface and in fractures of zircons found in sedimentary sources.[1]
More recent studies have also shown that there are additional unknown factors that contribute to Ti incorporation in zircons. The chemical activity of SiO2, pressure variance, disequilibrium crystallization from melts, late crystal growth in hydrous melts, or non-Henry's Law substitution in zircon crystals all may play a role in altering predicted crystallization temperatures.[11]
References
[edit]
References
[edit]- ^ a b c d e f Watson, E.B.; Wark, D.A.; Thomas, J.B. (3 March 2006). "Crystallization thermometers for zircon and rutile". Contributions to Mineral Petrology. 151: 413–433. doi:10.1007/s00410-006-0068-5.
- ^ a b c d Watson, E.B.; Harrison, T.M. (6 May 2005). "Zircon Thermometer Reveals Minimum Melting Conditions on Earliest Earth". Science Magazine. 308: 841–843. doi:10.1126/science.1110873.
- ^ a b Finch, Robert J.; Hanchar, John M. "Structure and chemistry of zircon and zircon-group minerals". Reviews in Mineralogy and Geochemistry. 53 (1): 1-25. doi:10.2113/0530001.
{{cite journal}}
:|access-date=
requires|url=
(help); Check date values in:|accessdate=
(help) - ^ Cherniak, D.J.; Hanchar, J.M.; Watson, E.B. "Rare earth diffusion in zircon". Chemical Geology. 134 (4): 289-301. doi:10.1016/S0009-2541(96)00098-8.
{{cite journal}}
:|access-date=
requires|url=
(help); Check date values in:|accessdate=
(help) - ^ Cherniak, D.J.; Hanchar, J.M.; Watson, E.B. "Diffusion of tetravalent cations in zircon". Contributions to Mineralogy and Petrology. 127 (4): 383-390. doi:10.1007/s004100050287.
{{cite journal}}
:|access-date=
requires|url=
(help); Check date values in:|accessdate=
(help) - ^ Cherniak, D.J.; Watson, E.B. "Pb diffusion in zircon". Chemical Geology. 172 (1–2): 5-24. doi:10.1016/S0009-2541(00)00233-3.
{{cite journal}}
:|access-date=
requires|url=
(help); Check date values in:|accessdate=
(help) - ^ Cherniak, D.J.; Watson, E.B (9 May 2007). "Ti diffusion in zircon". Chemical Geology. 242: 470–483. doi:10.1016/j.chemgeo.2007.05.005.
- ^ "SHRIMP Remote Operations System (SROS)". University of Milano-Bicocca. Retrieved 13 November 2014.
- ^ "Sample Preparation". SHRIMP RG Sample Preparation. USGS. Retrieved 8 Oct 2014.
- ^ Hoskin, P.W.; Schaltegger, Urs. "The composition of zircon and igneous and metamorphic petrogenesis". Reviews in Mineralogy and Geochemistry. 53 (1): 27–62. doi:10.2113/0530027.
- ^ Fu, Bin; Page, F. Zeb; Cavosie, Aaron J.; Fournelle, John; Kita, Noriko T.; Lackey, Jade Star; Wilde, Simon A.; Valey, John W. (12 Feb 2008). "Ti-in-zircon thermometry: applications and limitations". Contributions to Mineralogy and Petrology. 156: 197-215. doi:10.1007/s00410-008-0281-5.
{{cite journal}}
:|access-date=
requires|url=
(help); Check date values in:|accessdate=
(help) - ^ Finch, Robert J.; Hanchar, John M. (Jan 2003). "Structure and Chemistry of Zircon and Zircon-Group Minerals". Reviews in Mineralogy and Geochemistry. 53 (1): 1-25. doi:10.2113/0530001. Retrieved 29 November 2014.
- ^ Hoskin, Paul W.; Schaltegger, Urs (Jan 2003). "The Composition of Zircon and Igneous and Metamorphic Petrogenesis". Reviews in Mineralogy and Geochemistry. 53 (1): 27-62. doi:10.2113/0530027. Retrieved 29 November 2014.
- ^ Thomas, J.B.; Bodnar, R.J.; Shimizu, N.; Chesner, C.A. (Jan 2003). "Melt Inclusions in Zircon". Reviews in Mineralogy and Geochemistry. 53 (1): 63-87. doi:10.2113/0530063. Retrieved 29 November 2014.
- ^ Hanchar, John M.; Watson, E. Bruce (Jan 2003). "Zircon Saturation Thermometry". Reviews in Mineralogy and Geochemistry. 53 (1): 89-112. doi:10.2113/0530089. Retrieved 29 November 2014.
- ^ Cherniak, Daniele; Watson, E. Bruce (Jan 2003). "Diffusion in Zircon". Reviews in Mineralogy and Geochemistry. 53 (1): 113-143. doi:10.2113/0530113. Retrieved 29 November 2014.
Titles
[edit]Titanium in Zircon Geothermometer
Zircon Titanium Geothermometer