Somatostatin inhibitor
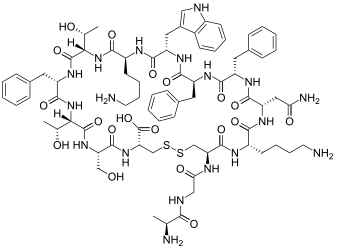
Somatostatin receptor antagonists (or somatostatin inhibitors) are a class of chemical compounds that work by imitating the structure of the neuropeptide somatostatin, which is an endogenous hormone found in the human body. The somatostatin receptors are G protein-coupled receptors. Somatostatin receptor subtypes in humans include sstr1, 2A, 2 B, 3, 4, and 5.[1] While normally expressed in the gastrointestinal (GI) tract, pancreas, hypothalamus, and central nervous system (CNS),[2] they are expressed in different types of tumours. The predominant subtype in cancer cells is the ssrt2 subtype, which is expressed in neuroblastomas, meningiomas, medulloblastomas, breast carcinomas, lymphomas, renal cell carcinomas, paragangliomas, small cell lung carcinomas, and hepatocellular carcinomas.[3]
As a radiopharmaceutical compound that is selective for somatostatin receptors, there is research being done for these radiolabeled compounds to act as diagnostic tests in PET scans for neuroendocrine tumors and other tumors not previously targeted with radiolabeled somatostatin receptor agonists, and to act as radiopharmaceutical therapeutic compound,[4] more specifically to conduct peptide radionuclide receptor therapy.
Some non-radiopharmaceutical compounds that are developed as competitive inhibitors of somatostatin, such as the hormone antagonist cyclosomatostatin.[5]
Somatostatin
[edit]
Somatostatin is a G protein-coupled receptor ligand. When the receptors are activated, it causes the cells where the receptors are expressed to decrease hormone secretion.[2] Mainly, as a neuroendocrine inhibitor, it exerts its effects on the gastrointestinal tract, pancreas, hypothalamus, and central nervous system,[2] causing hormone secretions coupled to this pathway to be reduced. It can affect neurotransmission and memory formation within the central nervous system.[2] Within human and animal models, it demonstrated its effects of preventing angiogenesis and reducing healthy and cancer cell proliferation.[2]
Within tumors, somatostatin receptors, mostly of the ssrt2 subtype, are expressed in most neuroendocrine tumors, breast tumors, some brain tumors, renal tumors, lymphomas, and prostate tumors.[3]
Somatostatin receptor antagonists in radiolabelling
[edit]These compounds work by binding to somatostatin receptors, which are more common in specific types of tumours. It does not activate the receptor. Due to the radionuclide, it will appear on PET scans. The radiolabeled somatostatin receptor antagonists share the following structure.[6] The antagonist has a peptide moiety,[7] and is responsible for receptor recognition and antagonist activities. Nomenclature is based on Radionuclide-Chelator-Receptor Antagonist.
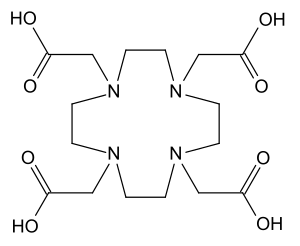
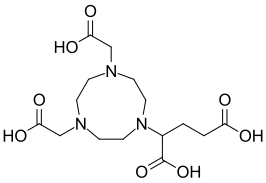
The structure of somatostatin receptor antagonists is similar to that of the agonists. Some agonists were already approved by the FDA for clinical use, such as In-DTPA-octreotide and Ga-DOTATATE.[4] Development started after the discovery of modifications that can be done to the octreotide group, an ssrt selective subtype agonist, to cause its agonistic effects to be lost and gain antagonistic effects.[8] Different subtype receptor antagonists were later developed.[9]
Research has been done mostly on the sstr2 receptor antagonist, as the sstr2 receptor is expressed on most tumors.[3] Somatostatin receptor antagonists are divided by generation based on the type of the subtype receptor antagonist. The first generation consists of sst2-ANT and BASS, which are sstr2 selective; and sst3-ODAN-8, which is selective for sstr3.[6]
After initial results of their increased sensitivity to neurocrine tumors appeared,[10][11] ssrt2 selective antagonists that had even higher affinity were developed.[12] These were LM3, JR10, and JR11, which make up the second generation.[6] JR11 was shown to be the most effective among these 3 antagonists, and compounds that entered further clinical development to act as a PET imaging agent or therapeutic agent carried this subtype antagonist.[6]
The presence of a chelator coupled to the subtype antagonist was shown to affect the biological properties.,[7][13][14][15] by increasing the binding stability of the radionuclide to the rest of the compound, and increasing the binding affinity to the receptor by allowing conjugation of the radionuclide to the receptor.[16] Compounds were developed with 3 macrocyclic chelators: DOTA, NODAGA, and CB-TE2A.[7] DOTA had already been used as a chelator in the radiolabeled somatostatin agonists,[7][17] as well as NODAGA[17] and CB-TE2A.[18] Ga-NODAGA-based compounds were shown to have a higher binding affinity than its DOTA analogues.[19] However, these somatostatin receptor antagonists showed a higher tumor uptake despite its lower affinity for ssrt receptors,[19] due to being able to bind a receptor despite its activation status.[10][20]
Compounds containing one of the radionuclides of indium-111, lutetium-177, copper-64, yttrium-80 and gallium-68 have been made. A study indicated the gallium compound had the lowest affinity to the sstr2 receptor.[19]
List of radiolabeled somatostatin receptor antagonists
[edit]The following listed compounds are those that have entered some phase of a pre-clinical study.[4]
Name | Status |
---|---|
Ga-DOTA-JR11 (Ga-OPS201)[4][6] | Pilot Study |
Ga-NODAGA-JR11 (Ga-OPS202)[4][21] | Phase 1/2 |
Lu-DOTA-JR11 (Lu-OPS201)[4][21] | Pilot Study |
In-DOTA-BASS[4] | Pilot Study |
In-DOTA-JR11[4] | Pilot Study |
The structure of the antagonist peptides shown in the above table are shown below.
Name | Structure |
---|---|
JR11 (sactreotide) | p-Cl-Phe1-cyclo(d-Cys2-Aph3(Hor)-d-Aph4(Cbm)-Lys5-Thr6-Cys7)d-Tyr8-NH2 |
BASS | p-NO2-Phe1-cyclo(d-Cys2-Tyr3-d-Trp4-Lys5-Thr6-Cys7)d-Tyr8-NH2 |
Further clinical studies of radiolabeled somatostatin receptor antagonists
[edit]Ga-NODAGA-JR11 had entered further clinical studies as an imaging agent,[6][22] while Lu-DOTA-JR11 had similar research done as a therapeutic agent,[6][22] as JR11 has a high binding affinity for ssrt2 subtype receptors which are highly expressed on the surface of tumor cells.[4] Gallium-containing agonists had already been established as an imaging agent. Lutetium-containing agonists were used as a therapeutic agent in peptide receptor radionuclide therapy,[6] due to the lower energy electrons emitted, and γ-emission causing easier dose adjustment to patient characteristics to avoid renal damage.[23] The NODAGA chelator was used over DOTA in Gallium antagonists due to higher binding affinity,[19] while no Lu-NODAGA compounds were developed due to established usage of Lu-DOTA derivative agonist drugs, and poor uptake compared to DOTA,[24] which is reverse that of the gallium-containing antagonists.
Safety of radiolabeled somatostatin receptor antagonists
[edit]In general, somatostatin receptor antagonists were noted to be well tolerated.[22] However, due to its mechanism of action, it may decrease the effectiveness of SSA therapy (Somatostatin Analogue Therapy),[22] but other studies indicate SSA may not need to be stopped if somatostatin antagonists are used to for tumor labelling instead of agonists. As somatostatin can cause inhibition of hormone production that uses it as a mediating hormone, it has an antiproliferative effect on cell tumors, especially in neuroendocrine tumors.[2] Somatostatin analogue therapy uses longer-acting agonists than the endogenous somatostatin to extend the antiproliferative effects.[25] Somatostatin receptor antagonists can bind to the receptors without activating them,[26] antagonizing the therapeutic inhibitory effects of SSA therapy.[26] Slow intravenous injection might be used until further safety data become available.[26]
Comparison of somatostatin receptor agonists and antagonists in radiolabelling
[edit]Agonists of the somatostatin receptor had been long established as an imaging agent, with the first agonist Ga-DOTATOC coming out in 2001,[27] which is based on a radiolabeled somatostatin receptor agonist drug octreotide, and further developments were based on its structure.[28] Agonists share the characteristic of being uptaken into tumor cells, and degraded intracellularly.[29] Antagonists, while not widely absorbed into the tumor cells, can bind to a wider range of receptors as they can bind to the receptors regardless if the receptors are activated or inactivated.[10][20] They thus are more sensitive to neuroendocrine tumors.[10][11]
Another study noted the antagonists showed lowered internalization into tumors, cleared from the blood quickly, and had a higher binding to tumors, which were noted to be properties benefitting its use over agonists in detecting metastatic tumors.[30]
A head-to-head study of the gallium-containing compounds, where the Ga-NODAGA-JR11 antagonist and Ga-DOTATOC agonist are directly compared, showed that Ga-NODAGA-JR11 had a higher hepatic metastatic tumor detection rate and lesion sensitivity than Ga-DOTATOC.[11]
Another head-to-head study of lutetium containing compound found the antagonist Lu-DOTA-JR11 bound with the receptors more quickly, had a longer retention time, and unbound more slowly than the Lu-DOTA-TATE agonist.[31]
Radiolabeled somatostatin receptor antagonists in Peptide Radionuclide Receptor Therapy (PRRT)
[edit]Somatostatin receptor antagonists are also being developed as therapeutic agents in peptide radionuclide receptor therapy (PRRT) due to the wider binding of antagonists compared to agonists.[23] Research indicated the antagonist Lu-DOTA-JR11 showed a higher tumor uptake, more double-strand breaks within tumor cells, longer adherence time to tumors and improved tumor-to-kidney dose ratio.[23][32][33][34]
Moreover, another study finds out the radionuclide terbium-161, which can release short-ranged electrons, can combine with somatostatin receptor antagonists that localize at the cell membrane. acting as an alternative to the current clinically used lutetium-somatostatin receptor agonist, which are localized at the cytoplasm and nucleus.[35] Moreover, Tb-antagonist in vitro shows 102-fold higher potency than Lu-antagonists in inhibiting tumor cell growth and survival prolongation in mice,[35] due to its high linear energy transfer.[36] This result is further repeated and confirmed in vivo,[35] showing the high potential and strengths of radiolabeled somatostatin receptor antagonists to treat neuroendocrine neoplasms.
Further potential
[edit]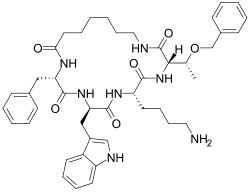
Other compounds other than radiolabelled somatostatin receptor antagonists have also been studied. Cyclosomatostatin is one such compound. Contrary to previously discussed compounds, cyclosomatostatin does not contain a radionuclide. It is a non-selective somatostatin receptor antagonist,[37] inhibiting the effects of somatostatin on target cells in the gastrointestinal tract, pancreas, hypothalamus, and central nervous system.[2] Cyclosomatostatin is used as a research chemical to investigate the effects of somatostatin on different cell types by antagonizing its receptors.[38] However it acts as an agonist in SH-SY5Y neuroblastoma cells.[37]
Cyclosomatostatin is also known by the following names:[5]
- 7-CPP
- antagonist SRIF-A
- CyCam
- cyclo(7-Ahep-Phe-Trp-Lys-Thr(Bzl))
- cyclo(7-aminoheptanoylphenylalanyl-tryptophyl-lysyl-benzylthreonyl)
- cyclo-(7-aminoheptanoyl-Phe-D-Trp-Lys-Thr(Bzl))
Cyclosomatostatin may have the possibility of treating complications of acute hemorrhage. Hepatic insulin sensitizing substance (HISS), a hormone, will be secreted by the liver which stimulates skeletal muscle glucose uptake when responding to insulin.[39] This action makes up around 56% of total insulin action.[39] Hemorrhage was shown to cause insulin resistance by this type of HISS-dependent insulin resistance (HDIR).[40] Two animal studies shows that cyclosomatostatin can help prevent HDIR without correcting the hyperglycemic condition in the situation of hemorrhage and exogenous somatostatin infusion.[41]
Cyclosomatostatin may be related to other indications, including the potential of blocking the suppression of gastric emptying triggered by corticotropin-releasing hormone (CRH),[42] the key regulator of the hypothalamic-pituitary-adrenal axis released to alter the body response caused by stress.[43] Furthermore, cyclosomatostatin, even if used alone, may modulate neurotransmitter levels. It increases acetylcholine (ACh) release by reversing the inhibitory effect of a substance, DHP agonist Bay K 8844, to L-type voltage-sensitive Ca2+ calcium channel.[44]
References
[edit]- ^ Taniyama, Yusuke; Suzuki, Takashi; Mikami, Yoshiki; Moriya, Takuya; Satomi, Susumu; Sasano, Hironobu (October 2005). "Systemic distribution of somatostatin receptor subtypes in human: an immunohistochemical study". Endocrine Journal. 52 (5): 605–611. doi:10.1507/endocrj.52.605. ISSN 0918-8959. PMID 16284440.
- ^ a b c d e f g O'Toole, Timothy J.; Sharma, Sandeep (2022), "Physiology, Somatostatin", StatPearls, Treasure Island (FL): StatPearls Publishing, PMID 30855911, retrieved 2023-03-14
- ^ a b c Reubi, Jean; Waser, Beatrice; Schaer, Jean-Claude; Laissue, Jean A. (2001-07-01). "Somatostatin receptor sst1–sst5 expression in normal and neoplastic human tissues using receptor autoradiography with subtype-selective ligands". European Journal of Nuclear Medicine. 28 (7): 836–846. doi:10.1007/s002590100541. ISSN 1619-7089. PMID 11504080. S2CID 8727308.
- ^ a b c d e f g h i Fani, Melpomeni; Nicolas, Guillaume P.; Wild, Damian (September 2017). "Somatostatin Receptor Antagonists for Imaging and Therapy". Journal of Nuclear Medicine. 58 (Suppl 2): 61S – 66S. doi:10.2967/jnumed.116.186783. ISSN 1535-5667. PMID 28864614. S2CID 9480322.
- ^ a b PubChem. "Cyclosomatostatin". pubchem.ncbi.nlm.nih.gov. Retrieved 2023-03-14.
- ^ a b c d e f g h Hou, Guozhu; Zhang, Yuwei; Liu, Yu; Wang, Peipei; Xia, Weibo; Xing, Xiaoping; Huo, Li; Li, Fang; Jing, Hongli (2022-02-24). "Head-to-Head Comparison of 68Ga-DOTA-TATE and 68Ga-DOTA-JR11 PET/CT in Patients With Tumor-Induced Osteomalacia: A Prospective Study". Frontiers in Oncology. 12: 811209. doi:10.3389/fonc.2022.811209. ISSN 2234-943X. PMC 8913035. PMID 35280786.
- ^ a b c d Fani, Melpomeni; Del Pozzo, Luigi; Abiraj, Keelara; Mansi, Rosalba; Tamma, Maria Luisa; Cescato, Renzo; Waser, Beatrice; Weber, Wolfgang A.; Reubi, Jean Claude; Maecke, Helmut R. (July 2011). "PET of somatostatin receptor-positive tumors using 64Cu- and 68Ga-somatostatin antagonists: the chelate makes the difference". Journal of Nuclear Medicine. 52 (7): 1110–1118. doi:10.2967/jnumed.111.087999. ISSN 1535-5667. PMID 21680701. S2CID 37563443.
- ^ Bass, R. T.; Buckwalter, B. L.; Patel, B. P.; Pausch, M. H.; Price, L. A.; Strnad, J.; Hadcock, J. R. (October 1996). "Identification and characterization of novel somatostatin antagonists". Molecular Pharmacology. 50 (4): 709–715. ISSN 0026-895X. PMID 8863814.
- ^ Reubi, J. C.; Schaer, J. C.; Wenger, S.; Hoeger, C.; Erchegyi, J.; Waser, B.; Rivier, J. (2000-12-05). "SST3-selective potent peptidic somatostatin receptor antagonists". Proceedings of the National Academy of Sciences of the United States of America. 97 (25): 13973–13978. Bibcode:2000PNAS...9713973R. doi:10.1073/pnas.250483897. ISSN 0027-8424. PMC 17685. PMID 11095748.
- ^ a b c d Fani, Melpomeni (2016-12-27), "Current and Future Radiopharmaceuticals in Neuroendocrine Tumor Imaging", Diagnostic and Therapeutic Nuclear Medicine for Neuroendocrine Tumors, Cham: Springer International Publishing, pp. 141–162, doi:10.1007/978-3-319-46038-3_7, ISBN 978-3-319-46036-9, retrieved 2023-03-14
- ^ a b c Nicolas, Guillaume P.; Schreiter, Nils; Kaul, Felix; Uiters, John; Bouterfa, Hakim; Kaufmann, Jens; Erlanger, Tobias E.; Cathomas, Richard; Christ, Emanuel; Fani, Melpomeni; Wild, Damian (June 2018). "Sensitivity Comparison of 68Ga-OPS202 and 68Ga-DOTATOC PET/CT in Patients with Gastroenteropancreatic Neuroendocrine Tumors: A Prospective Phase II Imaging Study". Journal of Nuclear Medicine. 59 (6): 915–921. doi:10.2967/jnumed.117.199760. ISSN 1535-5667. PMID 29191855. S2CID 40521791.
- ^ Cescato, Renzo; Erchegyi, Judith; Waser, Beatrice; Piccand, Véronique; Maecke, Helmut R.; Rivier, Jean E.; Reubi, Jean Claude (2008-07-10). "Design and in vitro characterization of highly sst2-selective somatostatin antagonists suitable for radiotargeting". Journal of Medicinal Chemistry. 51 (13): 4030–4037. doi:10.1021/jm701618q. ISSN 1520-4804. PMC 2789649. PMID 18543899.
- ^ Decristoforo, C.; Mather, S. J. (September 2002). "The influence of chelator on the pharmacokinetics of 99mTc-labelled peptides". The Quarterly Journal of Nuclear Medicine. 46 (3): 195–205. ISSN 1125-0135. PMID 12134136.
- ^ Rusckowski, M.; Qu, T.; Gupta, S.; Ley, A.; Hnatowich, D. J. (December 2001). "A comparison in monkeys of (99m)Tc labeled to a peptide by 4 methods". Journal of Nuclear Medicine. 42 (12): 1870–1877. ISSN 0161-5505. PMID 11752087.
- ^ Prasanphanich, Adam F.; Nanda, Prasant K.; Rold, Tammy L.; Ma, Lixin; Lewis, Michael R.; Garrison, Jered C.; Hoffman, Timothy J.; Sieckman, Gary L.; Figueroa, Said D.; Smith, Charles J. (2007-07-24). "[64Cu-NOTA-8-Aoc-BBN(7-14)NH2] targeting vector for positron-emission tomography imaging of gastrin-releasing peptide receptor-expressing tissues". Proceedings of the National Academy of Sciences of the United States of America. 104 (30): 12462–12467. Bibcode:2007PNAS..10412462P. doi:10.1073/pnas.0705347104. ISSN 0027-8424. PMC 1914305. PMID 17626788.
- ^ a b Eychenne, Romain; Bouvry, Christelle; Bourgeois, Mickael; Loyer, Pascal; Benoist, Eric; Lepareur, Nicolas (2020-09-20). "Overview of Radiolabeled Somatostatin Analogs for Cancer Imaging and Therapy". Molecules. 25 (17): 4012. doi:10.3390/molecules25174012. ISSN 1420-3049. PMC 7504749. PMID 32887456.
- ^ a b Ghosh, Sukhen C.; Pinkston, Kenneth L.; Robinson, Holly; Harvey, Barrett R.; Wilganowski, Nathaniel; Gore, Karen; Sevick-Muraca, Eva M.; Azhdarinia, Ali (February 2015). "Comparison of DOTA and NODAGA as chelators for (64)Cu-labeled immunoconjugates". Nuclear Medicine and Biology. 42 (2): 177–183. doi:10.1016/j.nucmedbio.2014.09.009. ISSN 1872-9614. PMID 25457653.
- ^ Wadas, Thaddeus J.; Anderson, Carolyn J. (2006). "Radiolabeling of TETA- and CB-TE2A-conjugated peptides with copper-64". Nature Protocols. 1 (6): 3062–3068. doi:10.1038/nprot.2006.431. ISSN 1750-2799. PMID 17406569. S2CID 2930589.
- ^ a b c d Fani, Melpomeni; Braun, Friederike; Waser, Beatrice; Beetschen, Karin; Cescato, Renzo; Erchegyi, Judit; Rivier, Jean E.; Weber, Wolfgang A.; Maecke, Helmut R.; Reubi, Jean Claude (September 2012). "Unexpected sensitivity of sst2 antagonists to N-terminal radiometal modifications". Journal of Nuclear Medicine. 53 (9): 1481–1489. doi:10.2967/jnumed.112.102764. ISSN 1535-5667. PMID 22851637. S2CID 6894025.
- ^ a b Carollo, Angela; Papi, Stefano; Grana, Chiara M.; Mansi, Luigi; Chinol, Marco (2019). "State of the Art and Recent Developments of Radiopharmaceuticals for Pancreatic Neuroendocrine Tumors Imaging". Current Radiopharmaceuticals. 12 (2): 107–125. doi:10.2174/1874471012666190306104450. ISSN 1874-4729. PMID 30843499. S2CID 73513507.
- ^ a b Reidy, Diane Lauren; Pandit-Taskar, Neeta; Krebs, Simone; O' Donoghue, Joseph A.; Raj, Nitya Prabhakar; Cruz, Elizabeth; Pham, Hanh; Lashley, Alicia; Bodei, Lisa; Weber, Wolfgang (2017-05-20). "Theranostic trial of well differentiated neuroendocrine tumors (NETs) with somatostatin antagonists 68Ga-OPS202 and 177Lu-OPS201". Journal of Clinical Oncology. 35 (15_suppl): 4094. doi:10.1200/jco.2017.35.15_suppl.4094. ISSN 0732-183X.
- ^ a b c d Pauwels, Elin; Cleeren, Frederik; Bormans, Guy; Deroose, Christophe M. (2018). "Somatostatin receptor PET ligands - the next generation for clinical practice". American Journal of Nuclear Medicine and Molecular Imaging. 8 (5): 311–331. ISSN 2160-8407. PMC 6261874. PMID 30510849.
- ^ a b c Ahmadi Bidakhvidi, Niloefar; Goffin, Karolien; Dekervel, Jeroen; Baete, Kristof; Nackaerts, Kristiaan; Clement, Paul; Van Cutsem, Eric; Verslype, Chris; Deroose, Christophe M. (2021-12-28). "Peptide Receptor Radionuclide Therapy Targeting the Somatostatin Receptor: Basic Principles, Clinical Applications and Optimization Strategies". Cancers. 14 (1): 129. doi:10.3390/cancers14010129. ISSN 2072-6694. PMC 8749814. PMID 35008293.
- ^ Mitran, Bogdan; Rinne, Sara S.; Konijnenberg, Mark W.; Maina, Theodosia; Nock, Berthold A.; Altai, Mohamed; Vorobyeva, Anzhelika; Larhed, Mats; Tolmachev, Vladimir; de Jong, Marion; Rosenström, Ulrika; Orlova, Anna (2019-12-15). "Trastuzumab cotreatment improves survival of mice with PC-3 prostate cancer xenografts treated with the GRPR antagonist 177 Lu-DOTAGA-PEG2 -RM26". International Journal of Cancer. 145 (12): 3347–3358. doi:10.1002/ijc.32401. ISSN 1097-0215. PMC 6852655. PMID 31077356.
- ^ Stueven, Anna Kathrin; Kayser, Antonin; Wetz, Christoph; Amthauer, Holger; Wree, Alexander; Tacke, Frank; Wiedenmann, Bertram; Roderburg, Christoph; Jann, Henning (2019-06-22). "Somatostatin Analogues in the Treatment of Neuroendocrine Tumors: Past, Present and Future". International Journal of Molecular Sciences. 20 (12): 3049. doi:10.3390/ijms20123049. ISSN 1422-0067. PMC 6627451. PMID 31234481.
- ^ a b c Bodei, Lisa; Weber, Wolfgang A. (June 2018). "Somatostatin Receptor Imaging of Neuroendocrine Tumors: From Agonists to Antagonists". Journal of Nuclear Medicine. 59 (6): 907–908. doi:10.2967/jnumed.117.205161. ISSN 1535-5667. PMID 29602817. S2CID 4497746.
- ^ Hofmann, M.; Maecke, H.; Börner, R.; Weckesser, E.; Schöffski, P.; Oei, L.; Schumacher, J.; Henze, M.; Heppeler, A.; Meyer, J.; Knapp, H. (December 2001). "Biokinetics and imaging with the somatostatin receptor PET radioligand (68)Ga-DOTATOC: preliminary data". European Journal of Nuclear Medicine. 28 (12): 1751–1757. doi:10.1007/s002590100639. ISSN 0340-6997. PMID 11734911. S2CID 22943690.
- ^ Helgebostad, Rasmus; Revheim, Mona-Elisabeth; Johnsrud, Kjersti; Amlie, Kristine; Alavi, Abass; Connelly, James Patrick (2022-02-18). "Clinical Applications of Somatostatin Receptor (Agonist) PET Tracers beyond Neuroendocrine Tumors". Diagnostics (Basel, Switzerland). 12 (2): 528. doi:10.3390/diagnostics12020528. ISSN 2075-4418. PMC 8870812. PMID 35204618.
- ^ "Somatostatin Receptor Antagonist - an overview | ScienceDirect Topics". www.sciencedirect.com. Retrieved 2023-03-14.
- ^ Park, Sonya; Parihar, Ashwin Singh; Bodei, Lisa; Hope, Thomas A.; Mallak, Nadine; Millo, Corina; Prasad, Kalpna; Wilson, Don; Zukotynski, Katherine; Mittra, Erik (October 2021). "Somatostatin Receptor Imaging and Theranostics: Current Practice and Future Prospects". Journal of Nuclear Medicine. 62 (10): 1323–1329. doi:10.2967/jnumed.120.251512. ISSN 1535-5667. PMC 9364764. PMID 34301785.
- ^ Mansi, Rosalba; Plas, Pascale; Vauquelin, Georges; Fani, Melpomeni (2021-12-04). "Distinct In Vitro Binding Profile of the Somatostatin Receptor Subtype 2 Antagonist [177Lu]Lu-OPS201 Compared to the Agonist [177Lu]Lu-DOTA-TATE". Pharmaceuticals (Basel, Switzerland). 14 (12): 1265. doi:10.3390/ph14121265. ISSN 1424-8247. PMC 8706879. PMID 34959665.
- ^ Dalm, Simone U.; Nonnekens, Julie; Doeswijk, Gabriela N.; de Blois, Erik; van Gent, Dik C.; Konijnenberg, Mark W.; de Jong, Marion (February 2016). "Comparison of the Therapeutic Response to Treatment with a 177Lu-Labeled Somatostatin Receptor Agonist and Antagonist in Preclinical Models". Journal of Nuclear Medicine. 57 (2): 260–265. doi:10.2967/jnumed.115.167007. ISSN 1535-5667. PMID 26514177. S2CID 29264191.
- ^ Nicolas, Guillaume P.; Mansi, Rosalba; McDougall, Lisa; Kaufmann, Jens; Bouterfa, Hakim; Wild, Damian; Fani, Melpomeni (September 2017). "Biodistribution, Pharmacokinetics, and Dosimetry of 177Lu-, 90Y-, and 111In-Labeled Somatostatin Receptor Antagonist OPS201 in Comparison to the Agonist 177Lu-DOTATATE: The Mass Effect". Journal of Nuclear Medicine. 58 (9): 1435–1441. doi:10.2967/jnumed.117.191684. ISSN 1535-5667. PMID 28450554. S2CID 42865787.
- ^ Wild, Damian; Fani, Melpomeni; Fischer, Richard; Del Pozzo, Luigi; Kaul, Felix; Krebs, Simone; Fischer, Richard; Rivier, Jean E. F.; Reubi, Jean Claude; Maecke, Helmut R.; Weber, Wolfgang A. (August 2014). "Comparison of somatostatin receptor agonist and antagonist for peptide receptor radionuclide therapy: a pilot study". Journal of Nuclear Medicine. 55 (8): 1248–1252. doi:10.2967/jnumed.114.138834. ISSN 1535-5667. PMID 24963127. S2CID 207407564.
- ^ a b c Borgna, Francesca; Haller, Stephanie; Rodriguez, Josep M. Monné; Ginj, Mihaela; Grundler, Pascal V.; Zeevaart, Jan Rijn; Köster, Ulli; Schibli, Roger; van der Meulen, Nicholas P.; Müller, Cristina (March 2022). "Combination of terbium-161 with somatostatin receptor antagonists-a potential paradigm shift for the treatment of neuroendocrine neoplasms". European Journal of Nuclear Medicine and Molecular Imaging. 49 (4): 1113–1126. doi:10.1007/s00259-021-05564-0. ISSN 1619-7089. PMC 8921065. PMID 34625828.
- ^ Hindié, Elif; Zanotti-Fregonara, Paolo; Quinto, Michele A.; Morgat, Clément; Champion, Christophe (May 2016). "Dose Deposits from 90Y, 177Lu, 111In, and 161Tb in Micrometastases of Various Sizes: Implications for Radiopharmaceutical Therapy". Journal of Nuclear Medicine. 57 (5): 759–764. doi:10.2967/jnumed.115.170423. hdl:11336/52884. ISSN 1535-5667. PMID 26912441. S2CID 3457261.
- ^ a b Stirnweis, Jörg; Boehmer, Frank-D.; Liebmann, Claus (August 2002). "The putative somatostatin antagonist, cyclo-(7-aminoheptanoyl-Phe-D-Trp-Lys-Thr[BZL]), may act as potent antiproliferative agonist". Peptides. 23 (8): 1503–1506. doi:10.1016/s0196-9781(02)00089-x. ISSN 0196-9781. PMID 12182954. S2CID 12988301.
- ^ Vilchis, C.; Bargas, J.; Pérez-Roselló, T.; Salgado, H.; Galarraga, E. (2002). "Somatostatin modulates Ca2+ currents in neostriatal neurons". Neuroscience. 109 (3): 555–567. doi:10.1016/s0306-4522(01)00503-6. ISSN 0306-4522. PMID 11823066. S2CID 40590426.
- ^ a b Lautt, W. W.; Macedo, M. P.; Sadri, P.; Takayama, S.; Duarte Ramos, F.; Legare, D. J. (July 2001). "Hepatic parasympathetic (HISS) control of insulin sensitivity determined by feeding and fasting". American Journal of Physiology. Gastrointestinal and Liver Physiology. 281 (1): G29–36. doi:10.1152/ajpgi.2001.281.1.G29. ISSN 0193-1857. PMID 11408252. S2CID 14651937.
- ^ Seredycz, Larissa I.; Ming, Zhi; Lautt, W. Wayne (November 2006). "Acute hemorrhage causes hepatic insulin sensitizing substance (HISS)-dependent insulin resistance". Canadian Journal of Physiology and Pharmacology. 84 (11): 1145–1151. doi:10.1139/y06-064. ISSN 0008-4212. PMID 17218979.
- ^ Seredycz, Larissa I.; Lautt, W. Wayne (2006). "Hemorrhage results in hepatic insulin-sensitizing substance-dependent insulin resistance mediated by somatostatin in rats". Neuroendocrinology. 84 (2): 94–102. doi:10.1159/000097484. ISSN 0028-3835. PMID 17202832. S2CID 24993789.
- ^ Smedh, U.; Kaplan, J. M.; Uvnäs-Moberg, K. (1999-01-22). "Corticotropin-releasing factor-induced suppression of gastric emptying in the rat is blocked by cyclo(7-aminoheptanoyl-phe-D-TRP-LYS-THR[BZL], an in vivo somatostatin antagonist". Neuroscience Letters. 260 (1): 41–44. doi:10.1016/s0304-3940(98)00953-7. ISSN 0304-3940. PMID 10027695. S2CID 24037692.
- ^ Stengel, Andreas; Taché, Yvette (October 2010). "Corticotropin-releasing factor signaling and visceral response to stress". Experimental Biology and Medicine. 235 (10): 1168–1178. doi:10.1258/ebm.2010.009347. ISSN 1535-3699. PMC 3169435. PMID 20881321.
- ^ Gray, D. B.; Zelazny, D.; Manthay, N.; Pilar, G. (August 1990). "Endogenous modulation of ACh release by somatostatin and the differential roles of Ca2+ channels". The Journal of Neuroscience. 10 (8): 2687–2698. doi:10.1523/JNEUROSCI.10-08-02687.1990. ISSN 0270-6474. PMC 6570278. PMID 1696982.