Plutonium-238
![]() Plutonium-238 oxide pellet glowing from its decay heat | |
General | |
---|---|
Symbol | 238Pu |
Names | plutonium-238, 238Pu, Pu-238 |
Protons (Z) | 94 |
Neutrons (N) | 144 |
Nuclide data | |
Half-life (t1/2) | 87.7 years[1][2] |
Isotope mass | 238.049553 Da |
Spin | 0 |
Parent isotopes | 242Cm (α) 238Np (β−) 238Am (β+) |
Decay products | 234U |
Decay modes | |
Decay mode | Decay energy (MeV) |
Alpha decay | 5.593 |
Isotopes of plutonium Complete table of nuclides |
Plutonium-238 (238
Pu
or Pu-238) is a radioactive isotope of plutonium that has a half-life of 87.7 years.
Plutonium-238 is a very powerful alpha emitter; as alpha particles are easily blocked, this makes the plutonium-238 isotope suitable for usage in radioisotope thermoelectric generators (RTGs) and radioisotope heater units. The density of plutonium-238 at room temperature is about 19.8 g/cc.[3] The material will generate about 0.57 watts per gram of 238Pu.[4]
The bare sphere critical mass of metallic plutonium-238 is not precisely known, but its calculated range is between 9.04 and 10.07 kilograms.[5]
History
[edit]Initial production
[edit]Plutonium-238 was the first isotope of plutonium to be discovered. It was synthesized by Glenn Seaborg and associates in December 1940 by bombarding uranium-238 with deuterons, creating neptunium-238.
238
92U
+ 2
1H
→ 238
93Np
+ 2
n
The neptunium isotope then undergoes β− decay to plutonium-238, with a half-life of 2.12 days:[6]
238
93Np
→ 238
94Pu
+
e−
+
ν
e
Plutonium-238 naturally decays to uranium-234 and then further along the radium series to lead-206. Historically, most plutonium-238 has been produced by Savannah River in their weapons reactor, by irradiating neptunium-237 (half life 2.144 Ma) with neutrons.[7]
237
93Np
+
n
→ 238
93Np
Neptunium-237 is a by-product of the production of plutonium-239 weapons-grade material, and when the site was shut down in 1988, 238Pu was mixed with about 16% 239Pu.[8]
Manhattan Project
[edit]
Plutonium was first synthesized in 1940 and isolated in 1941 by chemists at the University of California, Berkeley.[9][10]
The Manhattan Project began shortly after the discovery, with most early research (pre-1944) carried out using small samples manufactured using the large cyclotrons at the Berkeley Rad Lab and Washington University in St. Louis.[11]
Much of the difficulty encountered during the Manhattan Project regarded the production and testing of nuclear fuel. Both uranium and plutonium were eventually determined to be fissile, but in each case they had to be purified to select for the isotopes suitable for an atomic bomb.[12]
With World War II underway, the research teams were pressed for time. Micrograms of plutonium were made by cyclotrons in 1942 and 1943. In the fall of 1943 Robert Oppenheimer is quoted as saying "there's only a twentieth of a milligram in existence."[11]
By his request, the Rad Lab at Berkeley made available 1.2 mg of plutonium by the end of October 1943, most of which was taken to Los Alamos for theoretical work there.[11]
The world's second reactor, the X-10 Graphite Reactor built at a secret site at Oak Ridge, would be fully operational in 1944. In November 1943, shortly after its initial start-up, it was able to produce a minuscule 500 mg. However, this plutonium was mixed with large amounts of uranium fuel and destined for the nearby chemical processing pilot plant for isotopic separation (enrichment). Gram amounts of plutonium would not be available until spring of 1944.[13]
Industrial-scale production of plutonium only began in March 1945 when the B Reactor at the Hanford Site began operation.[12]
Plutonium-238 and human experimentation
[edit]While samples of plutonium were available in small quantities and being handled by researchers, no one knew what health effects this might have.[12]
Plutonium handling mishaps occurred in 1944, causing alarm in the Manhattan Project leadership as contamination inside and outside the laboratories was becoming an issue.[12] In August 1944, chemist Donald Mastick was sprayed in the face with a solution of plutonium chloride, causing him to accidentally swallow some.[12][14] Nose swipes taken of plutonium researchers indicated that plutonium was being breathed in.[12][15] Lead Manhattan Project chemist Glenn Seaborg, discoverer of many transuranium elements including plutonium, urged that a safety program be developed for plutonium research. In a memo to Robert Stone at the Chicago Met Lab, Seaborg wrote "that a program to trace the course of plutonium in the body be initiated as soon as possible ... [with] the very highest priority."[16] This memo was dated January 5, 1944, prior to many of the contamination events of 1944 in Building D where Mastick worked.[11] Seaborg later claimed that he did not at all intend to imply human experimentation in this memo, nor did he learn of its use in humans until far later due to the compartmentalization of classified information.[11]
With bomb-grade enriched plutonium-239 destined for critical research and for atomic weapon production, plutonium-238 was used in early medical experiments as it is unusable as atomic weapon fuel. However, 238Pu is far more dangerous than 239Pu due to its short half-life and being a strong alpha-emitter. It was soon found that plutonium was being excreted at a very slow rate, accumulating in test subjects involved in early human experimentation. This led to severe health consequences for the patients involved.
From April 10, 1945, to July 18, 1947, eighteen people were injected with plutonium as part of the Manhattan Project. Doses administered ranged from 0.095 to 5.9 microcuries (μCi).[12]
Albert Stevens, after a (mistaken) terminal cancer diagnosis which seemed to include many organs, was injected in 1945 with plutonium without his informed consent. He was referred to as patient CAL-1 and the plutonium consisted of 3.5 μCi 238Pu, and 0.046 μCi 239Pu, giving him an initial body burden of 3.546 μCi (131 kBq) total activity.[17][12] The fact that he had the highly radioactive plutonium-238 (produced in the 60-inch cyclotron at the Crocker Laboratory by deuteron bombardment of natural uranium)[17] contributed heavily to his long-term dose. Had all of the plutonium given to Stevens been the long-lived 239Pu as used in similar experiments of the time, Stevens's lifetime dose would have been significantly smaller. The short half-life of 87.7 years of 238Pu means that a large amount of it decayed during its time inside his body, especially when compared to the 24,100 year half-life of 239Pu.
After his initial "cancer" surgery removed many non-cancerous "tumors", Stevens survived for about 20 years after his experimental dose of plutonium before succumbing to heart disease; he had received the highest known accumulated radiation dose of any human patient.[11] Modern calculations of his lifetime absorbed dose give a significant 64 Sv (6400 rem) total.[11]
Weapons
[edit]The first application of 238Pu was its use in nuclear weapon components made at Mound Laboratories for Lawrence Radiation Laboratory (now Lawrence Livermore National Laboratory). Mound was chosen for this work because of its experience in producing the polonium-210-fueled Urchin initiator and its work with several heavy elements in a Reactor Fuels program. Two Mound scientists spent 1959 at Lawrence in joint development while the Special Metallurgical Building was constructed at Mound to house the project. Meanwhile, the first sample of 238Pu came to Mound in 1959.[18]
The weapons project called for the production of about 1 kg/year of 238Pu over a 3-year period. However, the 238Pu component[clarification needed] could not be produced to the specifications despite a 2-year effort beginning at Mound in mid-1961. A maximum effort was undertaken with 3 shifts a day, 6 days a week, and ramp-up of Savannah River's 238Pu production over the next three years to about 20 kg/year. A loosening of the specifications resulted in productivity of about 3%,[clarification needed] and production finally began in 1964.[citation needed]
Use in radioisotope thermoelectric generators
[edit]Beginning on January 1, 1957, Mound Laboratories RTG inventors Jordan & Birden were working on an Army Signal Corps contract (R-65-8- 998 11-SC-03-91) to conduct research on radioactive materials and thermocouples suitable for the direct conversion of heat to electrical energy using polonium-210 as the heat source.
In 1961, Capt. R. T. Carpenter had chosen 238Pu as the fuel for the first RTG (radioisotope thermoelectric generator) to be launched into space as auxiliary power for the Transit IV Navy navigational satellite. By January 21, 1963, the decision had yet to be made as to what isotope would be used to fuel the large RTGs for NASA programs.[19]
Early in 1964, Mound Laboratories scientists developed a different method of fabricating the weapon component that resulted in a production efficiency of around 98%.[20] This made available the excess Savannah River 238Pu production for Space Electric Power use just in time to meet the needs of the SNAP-27 RTG on the Moon, the Pioneer spacecraft, the Viking Mars landers, more Transit Navy navigation satellites (precursor to today's GPS) and two Voyager spacecraft, for which all of the 238Pu heat sources were fabricated at Mound Laboratories.[21]
The radioisotope heater units were used in space exploration beginning with the Apollo Radioisotope Heaters (ALRH) warming the Seismic Experiment placed on the Moon by the Apollo 11 mission and on several Moon and Mars rovers, to the 129 LWRHUs warming the experiments on the Galileo spacecraft.[22]
An addition to the Special Metallurgical building weapon component production facility was completed at the end of 1964 for 238Pu heat source fuel fabrication. A temporary fuel production facility was also installed in the Research Building in 1969 for Transit fuel fabrication. With completion of the weapons component project, the Special Metallurgical Building, nicknamed "Snake Mountain" because of the difficulties encountered in handling large quantities of 238Pu, ceased operations on June 30, 1968, with 238Pu operations taken over by the new Plutonium Processing Building,[where?] especially designed and constructed for handling large quantities of 238Pu. Plutonium-238 is given the highest relative hazard number (152) of all 256 radionuclides evaluated by Karl Z. Morgan et al. in 1963.[23]
Nuclear powered pacemakers
[edit]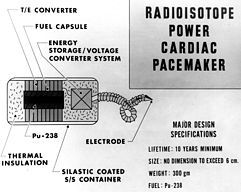
In the United States, when plutonium-238 became available for non-military uses, numerous applications were proposed and tested, including the cardiac pacemaker program that began on June 1, 1966, in conjunction with NUMEC.[24] The last of these units was implanted in 1988, as lithium-powered pacemakers, which had an expected lifespan of 10 or more years without the disadvantages of radiation concerns and regulatory hurdles, made these units obsolete.[25]
As of 2007[update], there were nine living people with nuclear-powered pacemakers in the United States, out of an original 139 recipients.[26] When these individuals die, the pacemaker is supposed to be removed and shipped to Los Alamos where the plutonium will be recovered.[27]
In a letter to the New England Journal of Medicine discussing a woman who received a Numec NU-5 decades ago that is continuously operating, despite an original $5,000 price tag equivalent to $23,000 in 2007 dollars, the follow-up costs have been about $19,000 compared with $55,000 for a battery-powered pacemaker.[26]
Another nuclear powered pacemaker was the Medtronics “Laurens-Alcatel Model 9000”.[28] Approximately 1600 nuclear-powered cardiac pacemakers and/or battery assemblies have been located across the United States, and are eligible for recovery by the Off-Site Source Recovery Project (OSRP) Team at Los Alamos National Laboratory (LANL).[29]
Production
[edit]Reactor-grade plutonium from spent nuclear fuel contains various isotopes of plutonium. 238Pu makes up only one or two percent, but it may be responsible for much of the short-term decay heat because of its short half-life relative to other plutonium isotopes. Reactor-grade plutonium is not useful for producing 238Pu for RTGs because difficult isotopic separation would be needed.
Pure plutonium-238 is prepared by neutron irradiation of neptunium-237,[30] one of the minor actinides that can be recovered from spent nuclear fuel during reprocessing, or by the neutron irradiation of americium in a reactor.[31] The targets are purified chemically, including dissolution in nitric acid to extract the plutonium-238. A 100 kg sample of light water reactor fuel that has been irradiated for three years contains only about 700 grams (0.7% by weight) of neptunium-237, which must be extracted and purified. Significant amounts of pure 238Pu could also be produced in a thorium fuel cycle.[32]
In the US, the Department of Energy's Space and Defense Power Systems Initiative of the Office of Nuclear Energy processes 238Pu, maintains its storage, and develops, produces, transports and manages safety of radioisotope power and heating units for both space exploration and national security spacecraft.[33] As of March 2015, a total of 35 kilograms (77 pounds) of 238Pu was available for civil space uses. Out of the inventory, 17 kg (37 lb) remained in a condition meeting NASA specifications for power delivery. Some of this pool of 238Pu was used in a multi-mission radioisotope thermoelectric generator (MMRTG) for the 2020 Mars Rover mission and two additional MMRTGs for a notional 2024 NASA mission. 21 kg (46 lb) would remain after that, including approximately 4 kg (8.8 lb) just barely meeting the NASA specification. Since isotope content in the material is lost over time to radioactive decay while in storage, this stock could be brought up to NASA specifications by blending it with a smaller amount of freshly produced 238Pu with a higher content of the isotope, and therefore energy density.[34]
U.S. production ceases and resumes
[edit]The United States stopped producing bulk 238Pu with the closure of the Savannah River Site reactors in 1988.[35][36][37] Since 1993, all of the 238Pu used in American spacecraft has been purchased from Russia. In total, 16.5 kilograms (36 lb) have been purchased, but Russia is no longer producing 238Pu, and their own supply is reportedly running low.[38][39]
In February 2013, a small amount of 238Pu was successfully produced by Oak Ridge's High Flux Isotope Reactor,[40] and on December 22, 2015, they reported the production of 50 grams (1.8 ounces) of 238Pu.[41][42]
In March 2017, Ontario Power Generation (OPG) and its venture arm, Canadian Nuclear Partners, announced plans to produce 238Pu as a second source for NASA. Rods containing neptunium-237[43] will be fabricated by Pacific Northwest National Laboratory (PNNL) in Washington State and shipped to OPG's Darlington Nuclear Generating Station in Clarington, Ontario, Canada where they will be irradiated with neutrons inside the reactor's core to produce 238Pu.[44][45]
In January 2019, it was reported that some automated aspects of its production were implemented at Oak Ridge National Laboratory in Tennessee, that are expected to triple the number of plutonium pellets produced each week.[46] The production rate is now expected to increase from 80 pellets per week to about 275 pellets per week, for a total production of about 400 grams per year.[46] The goal now is to optimize and scale-up the processes in order to produce an average of 1.5 kg (3.3 lb) per year by 2025.[47][45]
Applications
[edit]The main application of 238Pu is as the heat source in radioisotope thermoelectric generators (RTGs). The RTG was invented in 1954 by Mound scientists Ken Jordan and John Birden, who were inducted into the National Inventors Hall of Fame in 2013.[48] They immediately produced a working prototype using a 210Po heat source, and on January 1, 1957, entered into an Army Signal Corps contract (R-65-8- 998 11-SC-03-91) to conduct research on radioactive materials and thermocouples suitable for the direct conversion of heat to electrical energy using polonium-210 as the heat source.
In 1966, a study reported by SAE International described the potential for the use of plutonium-238 in radioisotope power subsystems for applications in space. This study focused on employing power conversions through the Rankine cycle, Brayton cycle, thermoelectric conversion and thermionic conversion with plutonium-238 as the primary heating element. The heat supplied by the plutonium-238 heating element was consistent between the 400 °C and 1000 °C regime but future technology could reach an upper limit of 2000 °C, further increasing the efficiency of the power systems. The Rankine cycle study reported an efficiency between 15 and 19% with inlet turbine temperatures of 730 °C, whereas the Brayton cycle offered efficiency greater than 20% with an inlet temperature of 840 °C. Thermoelectric converters offered low efficiency (3-5%) but high reliability. Thermionic conversion could provide similar efficiencies to the Brayton cycle if proper conditions reached.[49]
RTG technology was first developed by Los Alamos National Laboratory during the 1960s and 1970s to provide radioisotope thermoelectric generator power for cardiac pacemakers. Of the 250 plutonium-powered pacemakers Medtronic manufactured, twenty-two were still in service more than twenty-five years later, a feat that no battery-powered pacemaker could achieve.[50]
This same RTG power technology has been used in spacecraft such as Pioneer 10 and 11, Voyager 1 and 2, Cassini–Huygens and New Horizons, and in other devices, such as the Mars Science Laboratory and Mars 2020 Perseverance Rover, for long-term nuclear power generation.[51]
See also
[edit]References
[edit]- ^ Rebuilding the supply of Pu-238. Oregon State University.
- ^ US restarts production of plutonium-238 to power space missions. David Szondy, New Atlas. 23 December 2015.
- ^ Calculated from the atomic weight and the atomic volume. The unit cell, containing 16 atoms, has a volume of 319.96 cubic Å, according to Siegfried S. Hecker (2000). "Plutonium and its alloys: from atoms to microstructure" (PDF). Los Alamos Science. 26: 331.. This gives a density for 238Pu of (1.66053906660×10−24g/dalton×238.0495599 daltons/atom×16 atoms/unit cell)/(319.96 Å3/unit cell × 10−24cc/Å3) or 19.8 g/cc.
- ^ Miotla, Dennis (April 21, 2008). "Assessment of Plutonium-238 production alternatives" (PDF). www.energy.gov. p. 3. Retrieved September 21, 2020.
- ^ A. Blanchard; et al. (1999). Updated Critical Mass Estimates for Plutonium-238 (WSRC-MS-99-00313) (Report). Savannah River Site.
- ^ "The Discovery and Isolation of Plutonium". 29 September 2014.
- ^ "Plutonium-238 Production for Space Exploration". Retrieved 15 July 2020.
- ^ "MLM-CF-67-1-71 Plutonium 238 Oxide Shipment No. 33" (PDF). 1966-12-30. Archived from the original (PDF) on 2016-08-16. Retrieved 2016-08-05.
- ^ Seaborg, Glenn T. "An Early History of LBNL: Elements 93 and 94". Advanced Computing for Science Department, Lawrence Berkeley National Laboratory. Archived from the original on November 5, 2014. Retrieved September 17, 2008.
- ^ Glenn T. Seaborg (September 1981). Plutonium Story. Actinides-1981 conference, Pacific Grove, CA, USA, 10 Sep 1981. Lawrence Berkeley Laboratory, University of California. LBL-13492, DE82 004551.
- ^ a b c d e f g Welsome, Eileen (1999). The Plutonium Files:America's Secret Medical Experiments in the Cold War. Dial Press. ISBN 978-0385314022. Retrieved 18 November 2012.
- ^ a b c d e f g h Moss, William; Eckhardt, Roger (1995). "The Human Plutonium Injection Experiments" (PDF). Los Alamos Science. Radiation Protection and the Human Radiation Experiments (23): 177–223. Retrieved 13 November 2012.
- ^ Hewlett, Richard G.; Anderson, Oscar E. (1962). The New World, 1939–1946 (PDF). University Park, Pennsylvania: Pennsylvania State University Press. ISBN 978-0-520-07186-5. OCLC 637004643. Retrieved 26 March 2013.
- ^ After immediate treatment including scrubbing, stomach pumping, and citrate chelation (see Donald Mastick), less than 1 microgram of plutonium remained in his body. He lived to the age of 87.
- ^ Plutonium in Man: A Twenty-Five Year Review, UCRL 20850, TID-4500 (58th Ed.), Patricia W. Durbin, 1971.
- ^ Final Report Archived 2013-02-24 at the Wayback Machine, Advisory Committee on Human Radiation Experiments, 1985
- ^ a b Rowland, R.E., and Durbin, P.W. Survival, causes of death, and estimated tissue doses in a group of human beings injected with plutonium. United States: N. p., 1975. Web.
- ^ "Little Known Pu Stories" (PDF). Archived from the original (PDF) on 2016-08-16. Retrieved 2016-08-05.
- ^ G. R. Grove to D. L. Scot (1963-01-21). "Trip Report" (PDF). Archived from the original (PDF) on 2016-08-16. Retrieved 2016-08-05.
- ^ "Final Safety Analysis Report, January 15, 1975 (MLM-ENG-105)".
- ^ Carol Craig. "RTG: A Source of Power; A History of the Radioisotopic Thermoelectric Generators Fueled at Mound (MLM-MU-82-72-0006)" (PDF). Archived from the original (PDF) on 2016-08-16.
- ^ Johnson, Ernest (October 1988). "Light-Weight Radioisotope Heater Unit Final Analysis Safety Report". www.osti.gov. doi:10.2172/6531256. Retrieved September 21, 2020.
- ^ Morgan, Karl Z.; Snyder, W. S.; Ford, M. R. (1964). "Relative Hazard of the Various Radioactive Materials". Health Physics. 10 (3): 151–169. doi:10.1097/00004032-196403000-00002. PMID 14126790.
- ^ "Cardiac Pacemaker" (PDF). Monsanto Research Corporation. Archived from the original (PDF) on 2016-08-16.
- ^ Doyle, D. John (April 2013). "The Rise and Fall of the Nuclear Pacemaker" (PDF). p. 13. Archived (PDF) from the original on 12 September 2023. Retrieved 12 September 2023.
- ^ a b Emery, Gene (19 December 2007). "Nuclear pacemaker still energized after 34 years". Reuters. Archived from the original on 26 January 2021.
- ^ "Plutonium Powered Pacemaker (1974)". Oak Ridge Associated Universities. 10 May 2011. Archived from the original on 2 March 2019.
- ^ "MedTech Memoirs: The Plutonium-Powered Pacemaker". Medical Design and Outsourcing. 2016-01-13. Archived from the original on 20 June 2023. Retrieved 2021-09-02.
- ^ "Le pacemaker atomique" [The atomic pacemaker]. www.dissident-media.org (in French). Archived from the original on 20 June 2023. Retrieved 2021-09-02.
- ^ Werner, J.E.; Barklay, C.D.; Bickford, W.E.; Lord, D.B. (2013). Summary of Plutonium-238 Production Alternatives: Analysis Final Report (PDF) (Report). Idaho National Laboratory. INL/EXT-13-28846.
- ^ "Process for producing ultra-pure ... - Google Patents". Retrieved 2011-09-19.
- ^ "NASA needs Pu-238 now. The Medical Community needs isotopes now. Report to Congress on the Extraction of Medical Isotopes from Uranium-233" (PDF). U.S. Department of Energy, Office of Nuclear Energy, Science and Technology, Office of Isotopes for Medical Science. March 2001. Archived from the original (PDF) on 2013-09-21. Retrieved 2013-09-21.
- ^ "Space and Defense Power Systems". US Department of Energy. Retrieved 2022-04-18.
- ^ Caponiti, Alice. "Space and Defense Power Systems Program Information Briefing" (PDF). Lunar and Planetary Institute. NASA. Retrieved 24 March 2015.
- ^ Steven D. Howe; Douglas Crawford; Jorge Navarro; Terry Ring. "Economical Production of Pu - 238: Feasibility Study" (PDF). Center for Space Nuclear Research. Archived from the original (PDF) on 2013-09-04. Retrieved 2013-03-19.
- ^ "Plutonium-238 Is Produced In America For The First Time In Almost 30 Years". Australian Popular Science. Archived from the original on 2020-08-06. Retrieved 2017-03-01.
- ^ "SRS - History Highlights". www.srs.gov. Retrieved 2017-11-30.
- ^ "Commonly Asked Questions About Radioisotope Power Systems" (PDF). Idaho National Laboratory. July 2005. Archived from the original (PDF) on September 28, 2011. Retrieved 2011-10-24.
- ^ "Plutonium-238 Production Project" (PDF). Department of Energy. 5 February 2011. Archived from the original (PDF) on February 3, 2012. Retrieved 2 July 2012.
- ^ Clark, Stephen (20 March 2013). "U.S. laboratory produces first plutonium in 25 years". Spaceflightnow. Retrieved 21 March 2013.
- ^ Walli, Ron (22 December 2015). "ORNL achieves milestone with plutonium-238 sample". Oak Ridge National Laboratory. Retrieved 22 December 2015.
- ^ Harvey, Chelsea (30 December 2015). "This is the fuel NASA needs to make it to the edge of the solar system - and beyond". The Washington Post. Retrieved 4 January 2016.
- ^ United States to ship neptunium to Canada as part of Pu-238 production. International Panel on Fissile Materials. 5 March 2017.
- ^ NASA Re-starts PU-238 Production at Two Sites, Neutron Bytes, March 5, 2017
- ^ a b NASA Doesn't Have Enough Nuclear Fuel For Its Deep Space Missions. Ethan Siegel, Forbes. 13 December 2018.
- ^ a b Scientists Are Automating Plutonium Production So NASA Can Explore Deep Space. Daniel Oberhaus, Motherboard. 9 January 2019.
- ^ Scientists Find a New Way To Create the Plutonium That Powers Deep Space Missions. David Grossman, Popular Mechanics. 9 January 2019.
- ^ National Inventors Hall of Fame - John Birden Archived 2016-09-17 at the Wayback Machine.
- ^ Mahefkey, Edward T.; Berganini, David F. (1966). "Radioisotope Power Subsystems for Space Application". SAE Transactions. 74: 555–565. ISSN 0096-736X. JSTOR 44554237.
- ^ Kathy DeLucas; Jim Foxx; Robert Nance (January–March 2005). "From heat sources to heart sources: Los Alamos made material for plutonium-powered pumper". Actinide Research Quarterly. Retrieved 2015-07-09.
- ^ Alexandra Witze, Nuclear power: Desperately seeking plutonium, NASA has 35 kg of 238Pu to power its deep-space missions - but that will not get it very far., Nature, 25 Nov 2014
External links
[edit]- Story of Seaborg's discovery of Pu-238, especially pages 34–35. Archived 2014-02-23 at the Wayback Machine
- NLM Hazardous Substances Databank – Plutonium, Radioactive